The Lab Professional’s Guide to Mechanical Properties of Solids
Understanding the mechanical properties of solids is more critical than ever in today's rapidly evolving technological landscape. Whether you're developing new pharmaceuticals, formulating innovative food products, or engineering cutting-edge materials, these properties hold the key to unlocking superior performance and pushing the boundaries of what's possible.
.png)
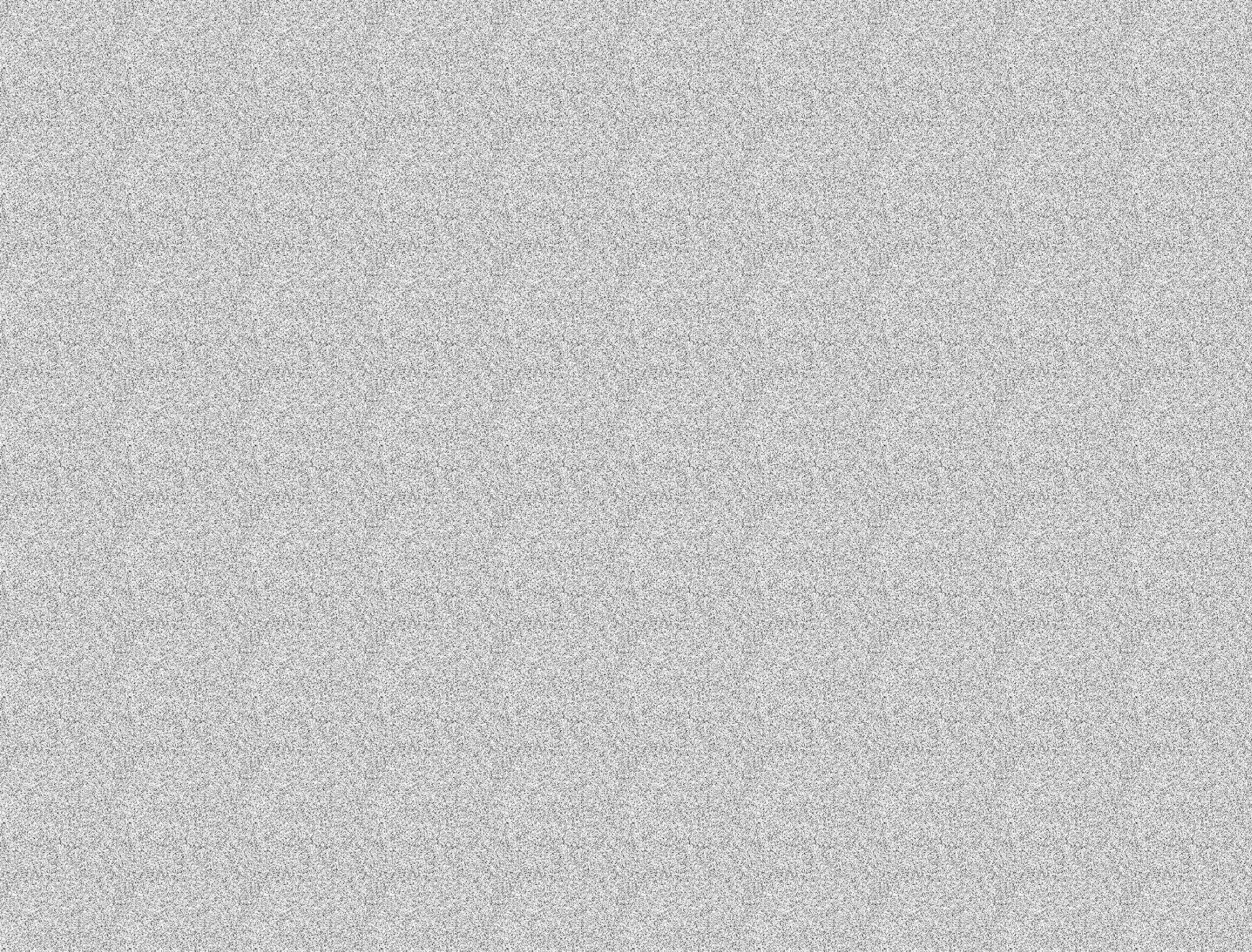
A recent report predicts that the global material testing market will increase from $6.4 billion in 2023 to $8.9 billion by 2032, with a significant portion driven by rising investment in research and development (R&D) and the growing demand for mechanical testing. This surge highlights the increasing emphasis on precision and reliability in material science across various industries.
Mechanical properties dictate how materials behave under stress, strain, and varying environmental conditions. Understanding these properties is essential for ensuring the quality, efficacy, and safety of products across various industries. In this guide, we'll explore the practical aspects of mechanical testing and provide valuable insights to help lab professionals optimize their workflows and achieve more accurate results.
What are the mechanical properties of solids?
The mechanical properties of solids define how materials react to external forces. Understanding these properties is essential for selecting suitable materials for any application because they directly affect performance, durability, and regulatory compliance. The key mechanical properties of solids include:
Tensile Strength
Tensile strength determines the ability of a material to withstand tension without breaking. This property is especially crucial in packaging, ensuring that materials can handle stress during transport and storage.
Elasticity
Elasticity refers to a material's ability to return to its original shape after being deformed. It is critical in industries where materials undergo various compressions, such as flexible packaging.
Hardness
The resistance of a material to indentation or scratching is known as hardness. Materials that are subjected to abrasive environments, such as in chemical processes, rely heavily on hardness.
Toughness
Toughness is a material's capacity to absorb energy and plastically deform without fracturing. Toughness is vital in industries like automotive and aerospace, where materials must absorb impacts and remain intact.
Fatigue Resistance
Fatigue resistance is a property measuring a material's ability to withstand repeated stress over time without failure. It is critical in industries like aerospace and energy, where materials are exposed to continuous stress cycles.
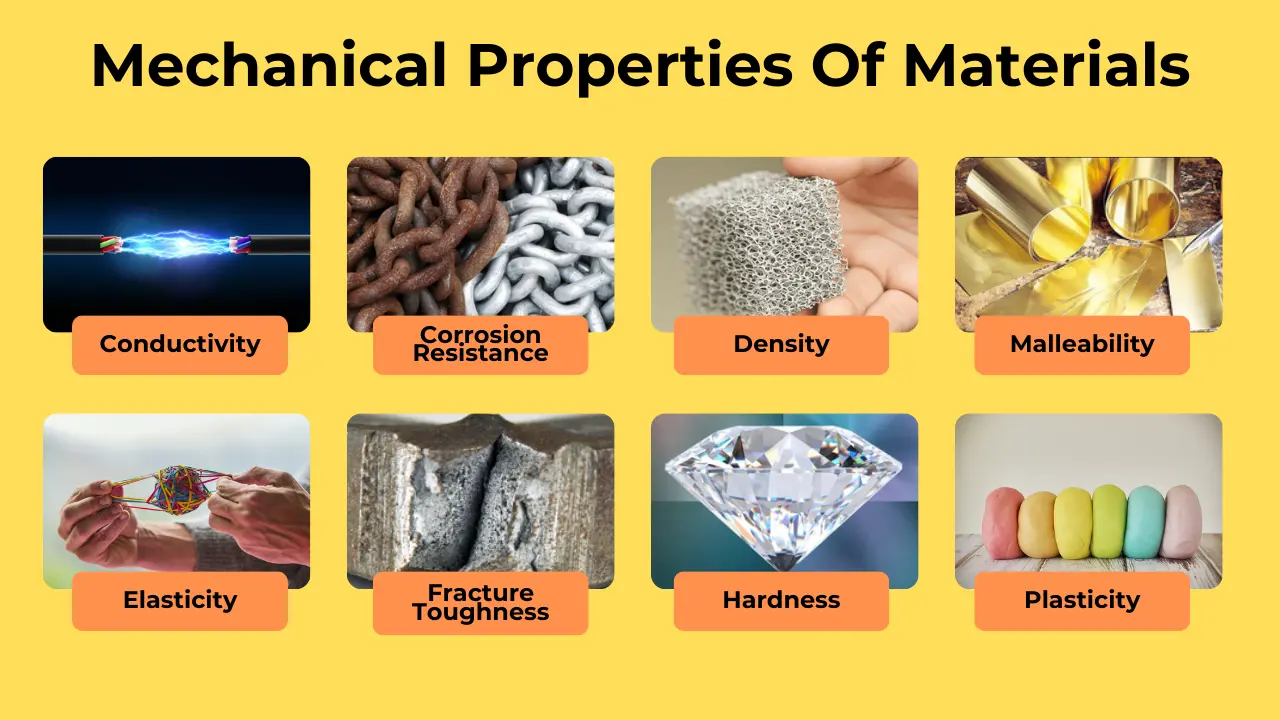
Why Understanding Mechanical Properties of Solids Matters in Lab Settings
In R&D settings, the mechanical properties of solids are a driving factor in product innovation, development, and durability. Lab professionals play a vital role in testing and evaluating these properties to ensure materials meet the required performance standards. Analyzing mechanical properties early in the R&D process allows labs to reduce trial-and-error cycles and accelerate time-to-market significantly.
For example, in the pharmaceutical industry, drug packaging must maintain integrity throughout the distribution chain, making tensile strength and toughness critical. In advanced materials, understanding properties such as elasticity and fatigue resistance ensures that components can endure the mechanical stresses they face, whether in aerospace applications or renewable energy systems.
Platforms like MaterialsZone provide detailed insights into these properties, which enables more efficient decision-making and faster product development. This helps labs optimize material performance while also ensuring compliance with increasingly stringent industry regulations.
Key Factors Affecting Mechanical Properties of Solids
Several factors influence the mechanical properties of materials, from their molecular composition to environmental conditions:
Internal Structure
The internal structure of a material, such as its crystalline arrangement, dictates how it will react to external forces. Metals with a face-centered cubic (FCC) structure, for example, tend to exhibit higher ductility than those with a body-centered cubic (BCC) structure, making them more suitable for applications requiring durability and flexibility.
Environmental Conditions
Environmental conditions such as temperature, pressure, and humidity can also alter a material's mechanical properties. For example, food and beverage industry packaging materials need to maintain their strength and elasticity at varying temperatures, especially during refrigeration. Similarly, in pharmaceutical applications, materials must resist temperature fluctuations to ensure that drugs remain safe and effective.
Manufacturing Processes
Manufacturing processes like heat treatment, forging, and extrusion can further modify a material's properties. Heat treatment, for example, can increase the hardness and strength of a metal by altering its crystalline structure. Lab professionals must account for these factors when selecting and testing materials, ensuring that the final product meets the desired performance standards.
Materials informatics and discovery platforms make it easier to track and analyze these factors in real-time. These powerful software solutions provide actionable insights to optimize material selection and performance, which reduces the number of iterations, accelerates materials R&D processes, and enhances efficiency.

6 Best Practices for Testing Mechanical Properties of Solids in the Lab
Accurately testing the mechanical properties of solids requires adherence to six best practices that ensure reliable and consistent results in lab settings:
1. Use Standardized Testing Protocols
Following industry standards like ASTM and ISO ensures that testing methods are consistent and that results are reliable.
These standards provide clear guidelines on how to prepare samples, conduct tests, and interpret results for various mechanical properties like tensile strength and hardness. Adhering to these standards allows for better comparison of data across different labs and research projects.
2. Select Appropriate Testing Methods
Different mechanical properties require different testing techniques. For instance, tensile strength is measured using tensile testers, which apply a controlled tension force until the material breaks, while hardness is gauged with tools such as the Vickers or Rockwell hardness tests, which measure the material's resistance to indentation.
Choosing the correct testing method is crucial for obtaining accurate and relevant data for the specific property of interest.
3. Regular Calibration of Testing Equipment
Testing equipment, such as tensile testers and hardness gauges, must be calibrated regularly to ensure they produce accurate results. Calibration involves comparing the equipment's measurements to known standards to identify and correct any deviations.
Regular calibration helps avoid errors caused by wear and tear, environmental factors, or other influences that can affect the equipment's accuracy.
4. Leverage Materials Informatics for Advanced Insights
Materials informatics platforms such as MaterialsZone enable lab professionals to integrate data from multiple sources, including mechanical testing results, chemical composition, and processing conditions.

Using AI and predictive algorithms, these platforms can analyze complex datasets to identify trends, predict material behavior, and optimize material selection. This approach can significantly reduce the time and resources required for traditional trial-and-error testing methods.
5. Automate Data Collection and Analysis
Automating the collection and analysis of mechanical property data not only reduces human error but also speeds up the testing process. Systems like MaterialsZone enable the automated capture of data from testing equipment, eliminating manual data entry and minimizing the risk of transcription errors.
Automated analysis tools can quickly process large volumes of cloud-stored data, identify outliers, and generate reports, freeing up lab professionals to focus on interpretation and decision-making.
6. Ensure Data Security and Collaboration
Protecting sensitive R&D data is critical, especially in industries like pharmaceuticals and advanced materials. Secure data management systems help prevent unauthorized access, accidental deletion, or data corruption. Implementing robust access controls and encryption protocols ensures data confidentiality and integrity.
Platforms that facilitate collaboration allow researchers to share data, insights, and results securely with colleagues and collaborators, promoting knowledge sharing and accelerating innovation.
Accelerating R&D with MaterialsZone
A deep understanding of mechanical properties plays a vital role in driving innovation and ensuring product quality in today's labs. From ensuring the integrity of pharmaceutical packaging to optimizing the performance of advanced materials, these properties are essential for meeting evolving industry demands.
MaterialsZone offers a comprehensive platform for managing and optimizing your mechanical testing processes. It allows labs to leverage powerful tools to gain a deeper understanding of material behavior, predict performance with greater accuracy, and ultimately reduce the risk of costly material failures. The platform fosters collaboration and ensures data integrity, enabling your team to make informed decisions with confidence.
Request a MaterialsZone demo to discover how it accelerates your R&D process and lets you bring innovative products to market faster.